Understanding and Mitigating Hydrogen Embrittlement in High-Strength Steel: A Practical Guide
- Tectron Blogs
- Mar 21
- 2 min read
Updated: Mar 27
Hydrogen embrittlement (HE) is a silent but devastating threat to high-strength steel components. It can lead to catastrophic failures, even under relatively low stress, and understanding its mechanisms and prevention is crucial for ensuring the reliability of critical parts. This guide provides a practical overview of HE, its causes, and effective mitigation strategies.
What is Hydrogen Embrittlement?
Hydrogen embrittlement occurs when hydrogen atoms diffuse into the steel's microstructure, weakening the bonds between iron atoms. This weakening can lead to cracking and fracture, particularly under tensile stress. High-strength steels are especially susceptible due to their inherent microstructure.
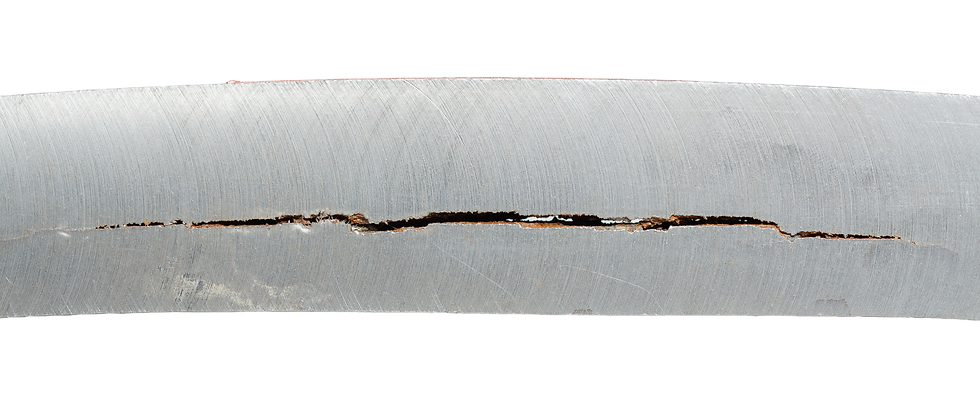
Key Factors Contributing to Hydrogen Embrittlement:
Hydrogen Sources:
Electroplating: Hydrogen is a byproduct of many plating processes.
Welding: Moisture in welding consumables or the atmosphere can introduce hydrogen.
Pickling and Acid Cleaning: Acids can release hydrogen during cleaning.
Corrosion: Hydrogen is a byproduct of electrochemical corrosion.
Wet Grinding: Grinding with coolant that contains water.
Steel Microstructure:
High-strength steels with martensitic or bainitic microstructures are more susceptible.
Inclusions and defects can act as hydrogen traps, increasing embrittlement.
Stress:
Tensile stress accelerates hydrogen diffusion and cracking.
Residual stresses from manufacturing processes can contribute.
Time and Temperature:
Hydrogen diffusion is time-dependent; longer exposure increases embrittlement.
Higher temperatures can accelerate diffusion, but also allow for hydrogen outgassing.
Practical Mitigation Strategies:
Process Control in Plating and Cleaning:
Use low-hydrogen plating processes.
Implement baking or heat treatment immediately after plating to drive out hydrogen.
Minimize pickling and acid cleaning times.
Use inhibitors in acid solutions to reduce hydrogen evolution.
Welding Best Practices:
Use low-hydrogen welding consumables.
Preheat and post-weld heat treatment to reduce hydrogen content.
Control arc length and minimize moisture exposure.
Use correct shielding gasses.
Corrosion Prevention:
Apply protective coatings to prevent corrosion.
Use corrosion-resistant alloys.
Control environmental conditions to minimize corrosion.
Properly design parts to avoid crevices where corrosion can start.
Heat Treatment Optimization:
Control heat treatment parameters to minimize residual stresses.
Temper high-strength steels to improve resistance to HE.
Vacuum heat treatment can minimize hydrogen introduction.
Material Selection:
Consider using alloys with lower susceptibility to HE.
Specify materials with controlled inclusion content.
Design Considerations:
Minimize stress concentrations in designs.
Avoid sharp corners and notches.
Use compressive residual stresses in critical areas.
Inspection and Testing:
Perform non-destructive testing (NDT) to detect cracks.
Conduct hydrogen content analysis on critical components.
Implement stress corrosion testing to evaluate material performance.
Environmental Control:
Control humidity in the work area.
Keep materials dry.
Control the temperature of coolants used during machining.
Baking and Dehydrogenation:
Implement baking procedures after processes that introduce hydrogen.
Ensure appropriate temperature and time for hydrogen outgassing.
Regular Training and Awareness:
Educate employees about the risks of hydrogen embrittlement.
Implement strict process control procedures.
Conclusion:
Hydrogen embrittlement poses a significant risk to high-strength steel components. By understanding its causes and implementing effective mitigation strategies, manufacturers can significantly reduce the likelihood of catastrophic failures. Careful process control, material selection, and design considerations are essential for ensuring the reliability and safety of high-strength steel applications.